The UW CTMR supports the development of exciting new muscle research through a pilot and feasibility grant program that allows investigators to obtain preliminary data needed to apply for follow-on funding and receive training in new skills. Funding up to $25,000 (direct costs) is available.
Applying
We are NOT currently accepting pilot applications. Please check back at the start of 2026 for another call for pilot applications. Information from our previous application call is included below for reference only.
If you are considering applying, reach out to CTMR Core Resource Directors & Personnel to discuss integrating the Cores into your proposal (a proposal requirement):
- MECHANICS & DEVICES CORE:
- Mike Regnier mregnier@uw.edu
- METABOLISM CORE:
- Dan Raftery draftery@uw.edu
- QUANTITATIVE ANALYSIS
- Matt Childers mcc7fb@uw.edu & Travis Tune ttune3@uw.edu
Current Awardees
Project Period: September 1, 2024 – March 31, 2025 (Year 6)
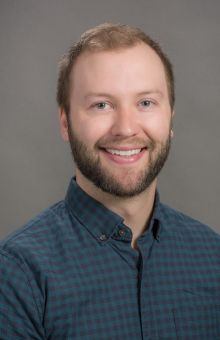
PI: Joe Powers, PhD, Assistant Professor Laboratory Medicine & Pathology Mechanical Engineering
Project: Measuring and modeling emergent mechanical behavior of remodeled myocytes due to Filamin C deletion
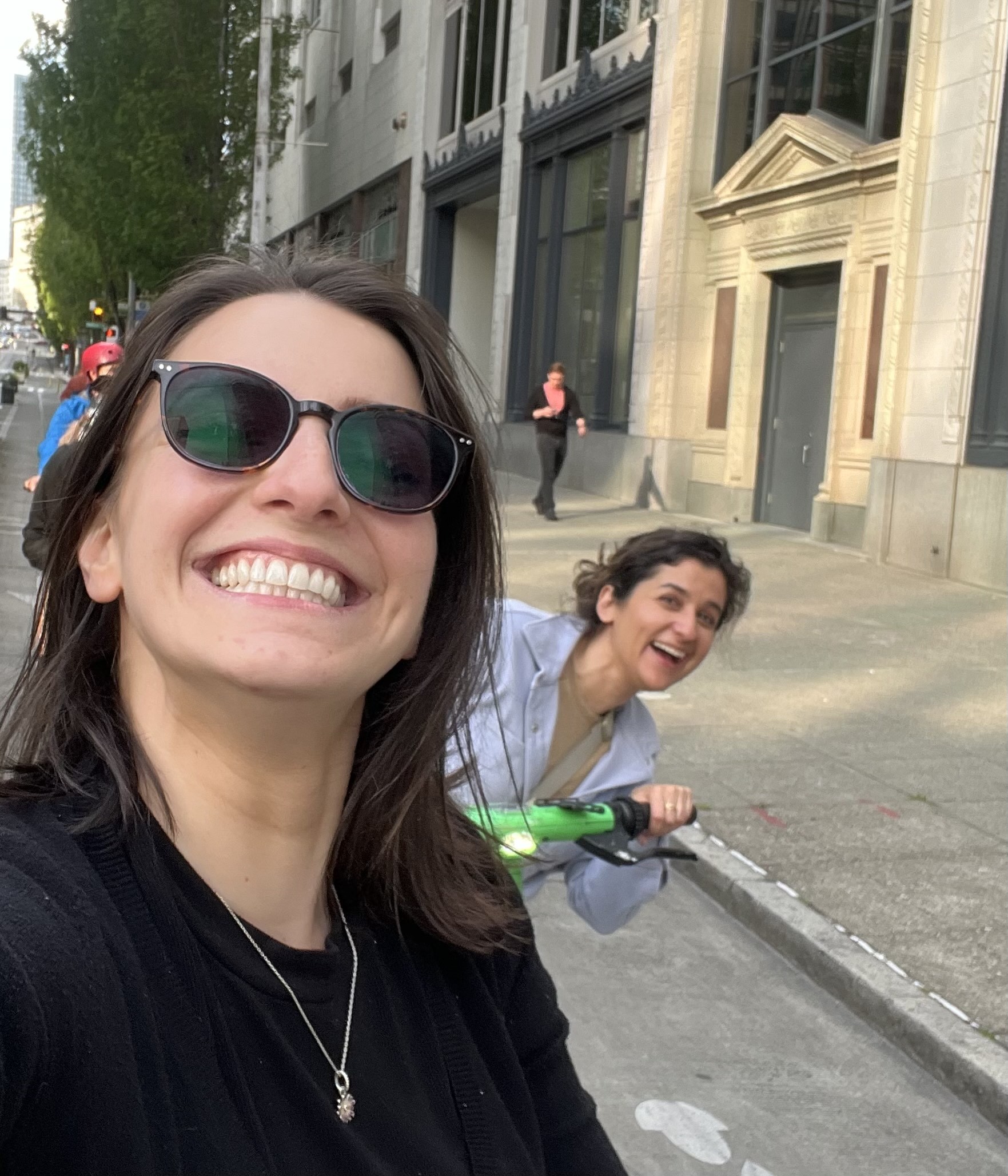
PI: Silvia Marchianò, PhD, PharmD, Acting Instructor, Department of Laboratory Medicine and Pathology & Elaheh Karbassi, PhD, Acting Instructor, Department of Laboratory Medicine and Pathology
Project: Mutational screening of ribonucleotide reductase activity using human pluripotent stem cell derived cardiomyocytes.
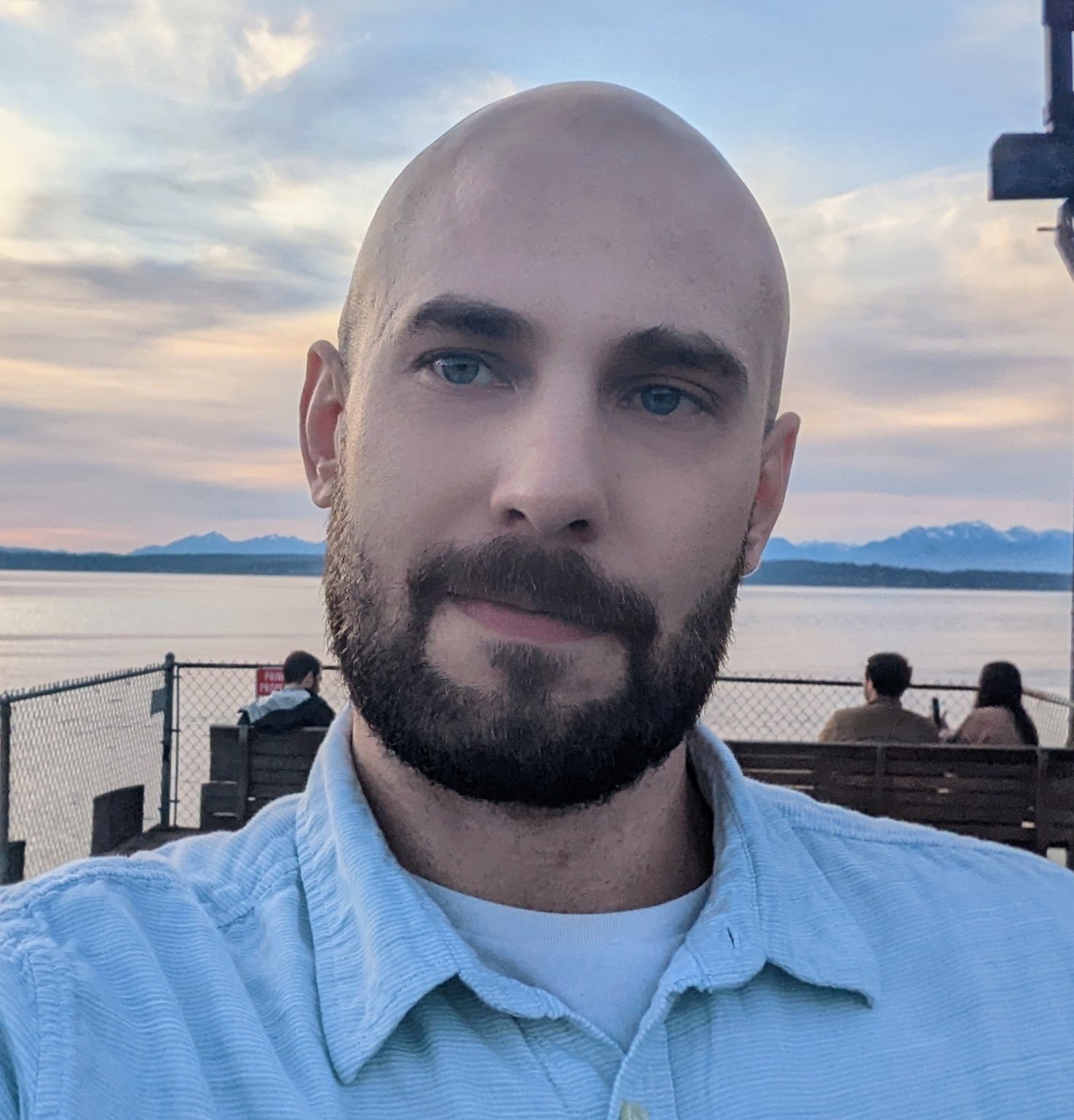
PI: Matthew Childers, Research Scientist 4, Department of Bioengineering
Project: Examining the Mechanisms of the Dilated Cardiomyopathy-Associated Myosin Mutation R369Q
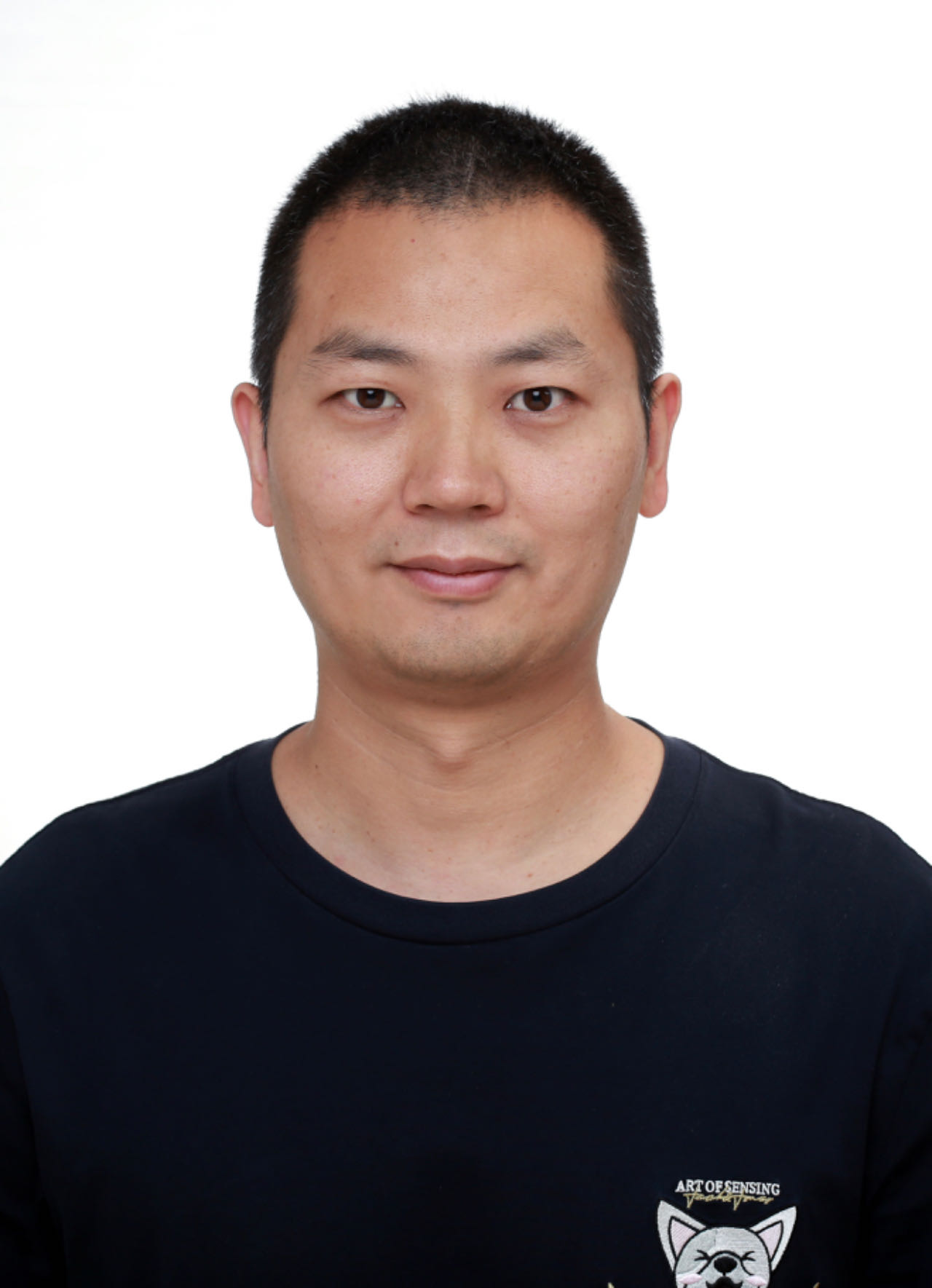
PI: Wentao Zhu, PhD, Acting Assistant Professor, Department of Anesthesiology & Pain Medicine
Project: A Comprehensive Metabolomics Investigation into the Molecular Mechanisms of ZFYVE1 in a Mouse Model of Heart Failure
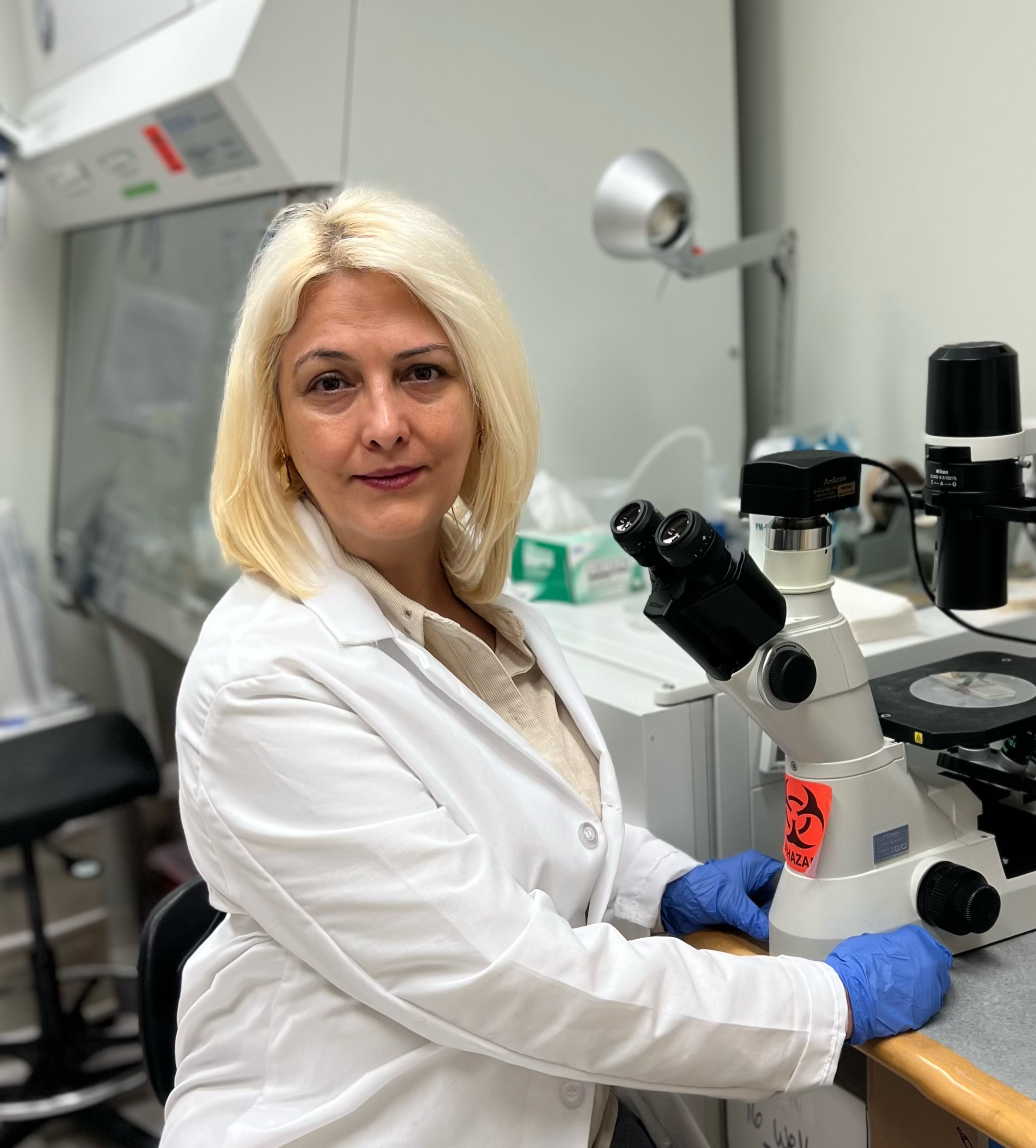
PI: Shabnam Salami, MD, MSc, FAHA, Acting Instructor, Department of Anesthesiology & Pain Medicine
Project: Mechanistic Insights into DOX-induced Accelerated Aging and Mitigation Strategies
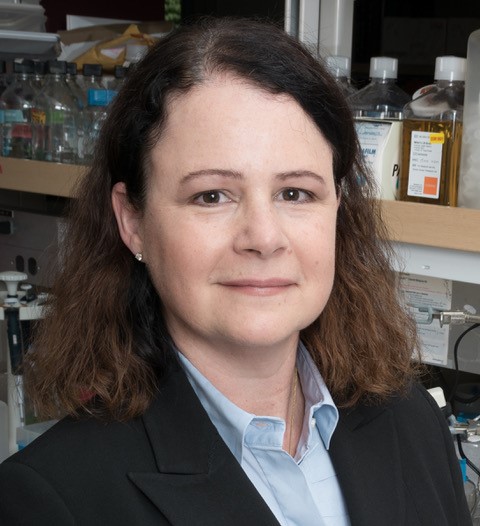

PI: Joel Chamberlain, PhD, Research Associate Professor, Division of Medical Genetics & Seung Wook Oh, PhD, Chief Operating Officer, Kinea Bio, Inc.
Project: A novel non-viral delivery of RNA therapeutics to treat myotonic dystrophy type 1 (DM1)
Previous Awardees
Project Period: May 1, 2023 – February 29, 2024 (Year 5)
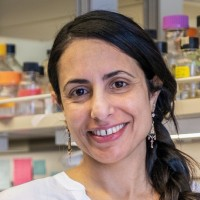
PI: Shiri Levy, PhD, Acting Instructor, Department of Biochemistry
Project: Epigenetic reprogramming of muscle stem cells in sarcopenia using AI-design computer protein binder
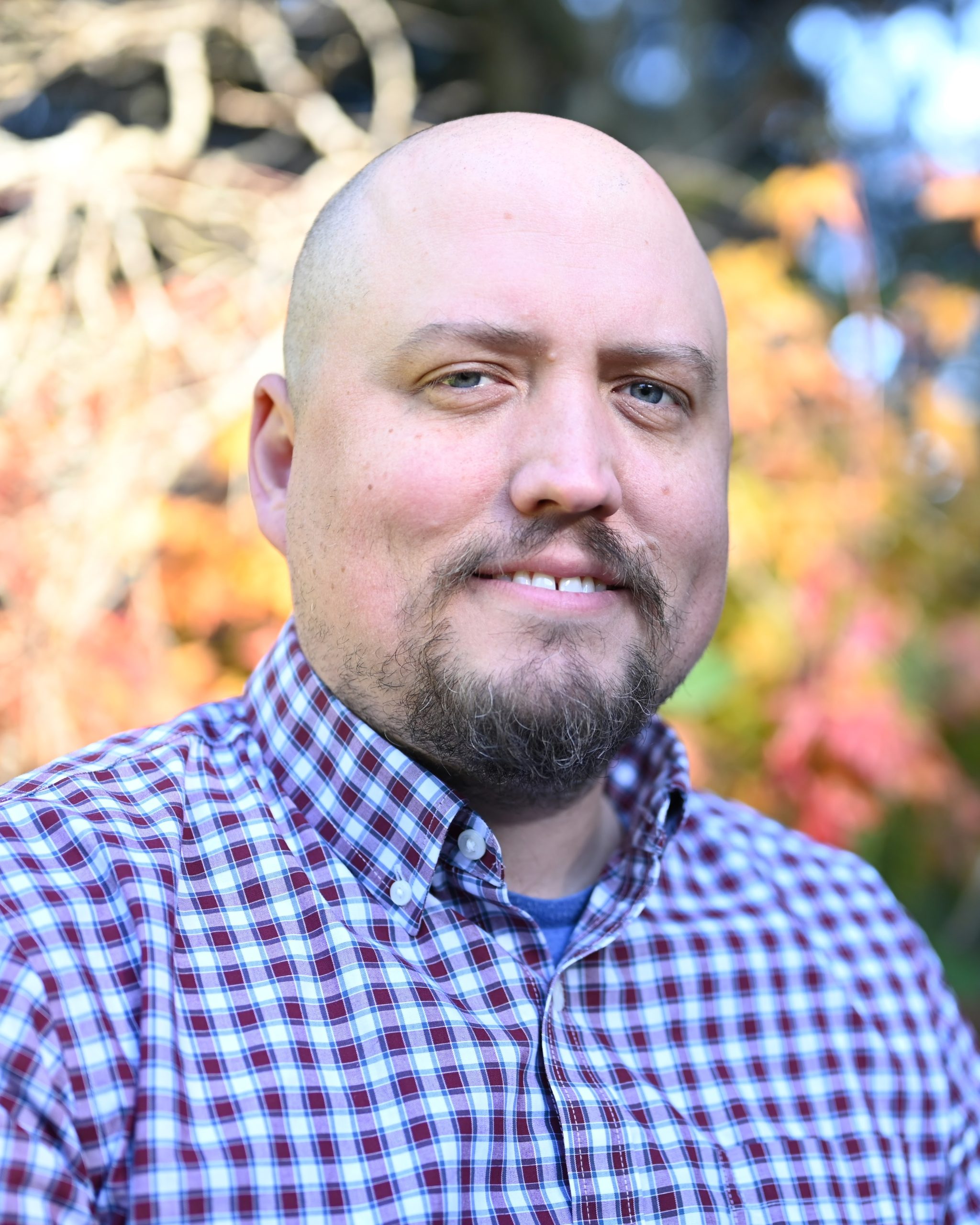
PI: Logan Murphy, PhD, Acting Instructor, Department of Physiology & Biophysics
Project: Sex-Dependent Recovery from Cervical Spinal Cord Injury with Electrical Spinal Stimulation Therapy: Role of Muscle Responses
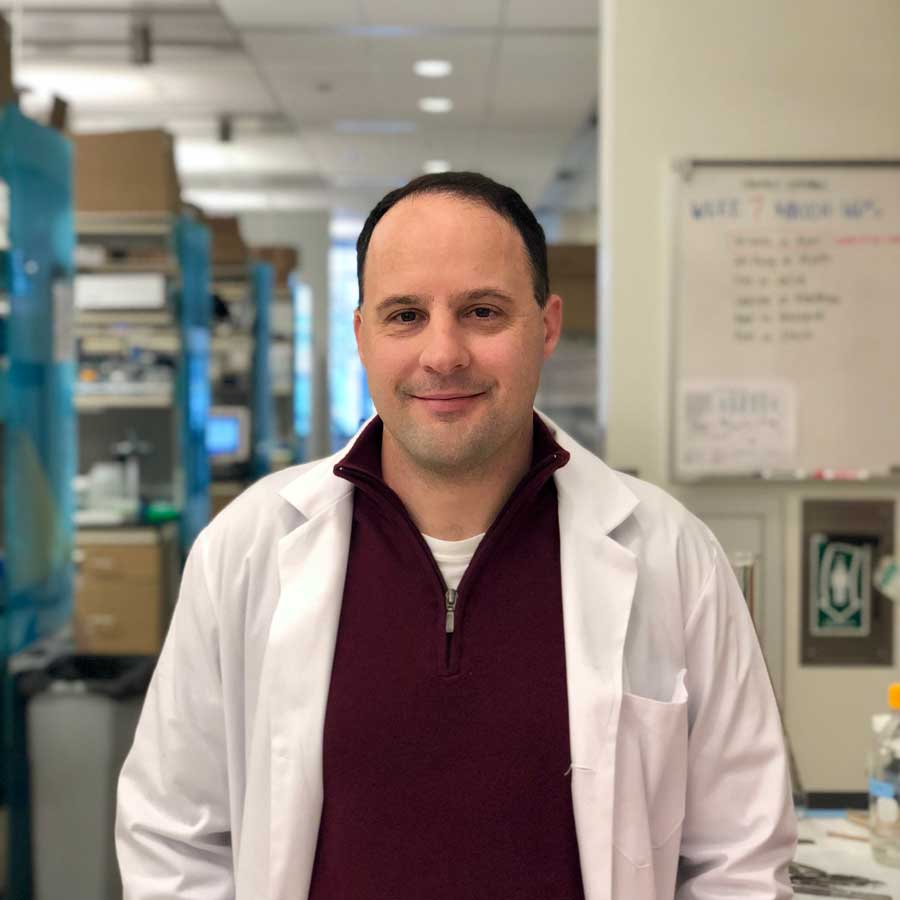
PI: Matthew Walker, PhD, Research Assistant Professor, Mitochondria and Metabolism Center & Department of Anesthesiology and Pain Medicine
Project: Computational approaches to study redox regulation of mitochondrial RNase P:
Implications for skeletal muscle mitochondrial biogenesis in heart failure
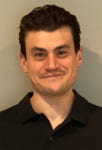
PI: Matthew Campbell, PhD, Acting Instructor, Department of Radiology
Project: Acute mitochondrial functional response to contraction in human skeletal myotube
Project Period: April 1, 2022 – February 28, 2023 (Year 4)
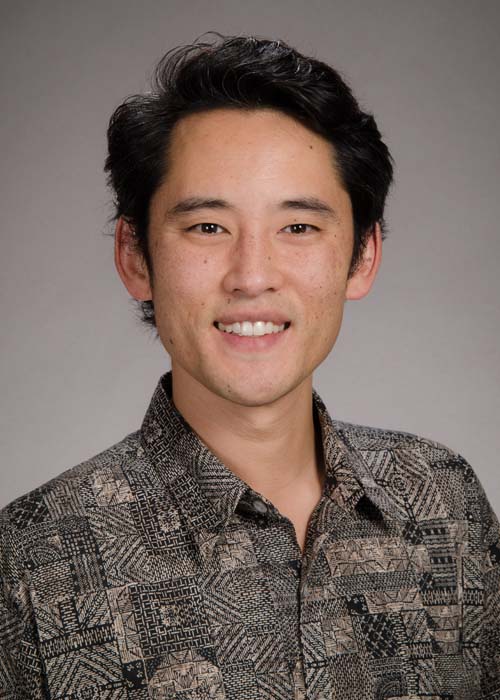
PI: Ronald Y. Kwon, Ph.D., Associate Professor, Department of Orthapaedics and Sports Medicine
Project: Genetic Determinants of Osteosarcopenia Risk
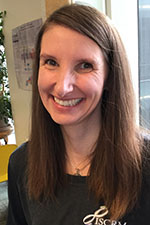
PI: Julie Mathieu, Ph.D., Assistant Professor, Comparative Medicine
Project: ANT1 function in induced pluripotent stem cell-derived cardiomyocytes
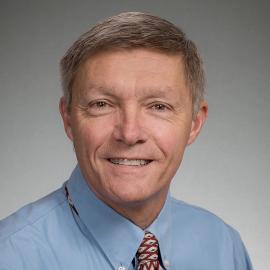
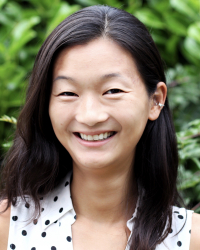
PI: David J Perkel, Ph.D., Professor, Departments of Biology and Otolaryngology; Bing W Brunton, Ph.D., Associate Professor, Department of Biology
Project: Measuring muscle performance in a vertebrate model of enhanced bipedal balance
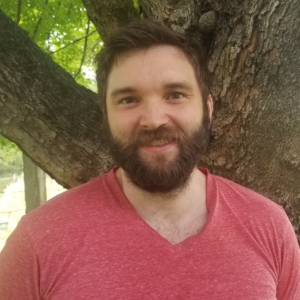
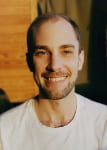
PI: Travis Tune, Ph.D., Research Scientist, Department of Biology; Matt Childers Ph.D., Postdoctoral Fellow, Department of Bioengineering
Project: Measuring muscle performance in a vertebrate model of enhanced bipedal balance
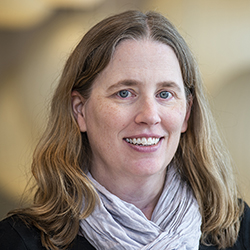
PI: Lisa Maves, Ph.D., Associate Professor, Pediatrics
Project: Quantitative Modeling to Determine Muscle Myosin Gene Orthology
Project Period: April 1, 2021-February 28, 2022 (Year 3)
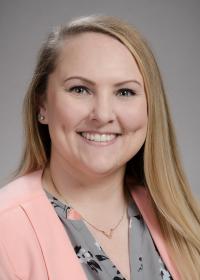
PI: Lindsey Anderson, Ph.D., Acting Instructor, Division of Gerontology and Geriatric Medicine
Project: Metabolomics approach to characterize the effects of androgen deprivation therapy on skeletal muscle in prostate cancer patients
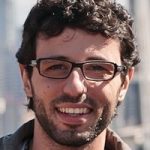
PI: Fausto Carnevale Neto, Ph.D., Acting Instructor, Department of Anesthesiology & Pain Medicine
Project: The role of mitochondrial metabolism on quiescent muscle stem cell proliferation and myogenesis
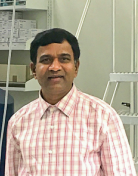
PI: G. A. Nagana Gowda, Ph.D., Research Associate Professor, Department of Anesthesiology & Pain Medicine
Project: Probing Energetics of Muscle Metabolism Using 31P NMR Metabolomics
Project Period: November 16, 2020 – February 28, 2021 (Year 2)
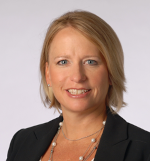
PI: Mary Beth Brown, Ph.D., Associate Professor, Department of Rehabilitation Medicine
Project: Metabolomics to reveal exercise adaptations and impact of gene therapy in a novel rat model of Duchene muscular dystrophy
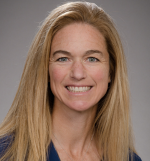
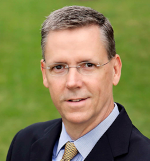
PIs: Jennifer Davis, Ph.D, Associate Professor, Departments of Lab Medicine & Pathology and Bioengineering; David Mack, Ph.D. Associate Professor, Department of Rehabilitation Medicine
Project: The role of MBNL1-Transcriptome Reprogramming In IPS-Skeletal Muscle Differentiation
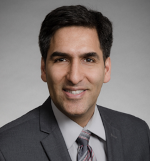
PI: Farid Moussavi-Harami, M.D., Assistant Professor, Division of Cardiology
Project: Metabolomic analysis of skeletal muscle in mouse dilated cardiomyopathy
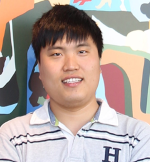
PI: Yuliang Wang, Ph.D., Research Assistant Professor, Paul G. Allen School of Computer Science & Engineering
Project: Identify metabolic interventions to mature iPSC-SkM by network-based multi-omic data integration
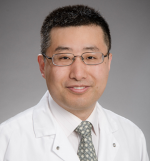
PI: Huiliang Zhang, Ph.D., Acting Instructor, Department of Laboratory Medicine & Pathology
Project: Mitochondrial function rejuvenation to restore aged heart muscle dysfunction
Project Period: April 1, 2020 – February 28, 2021 (Year 1)
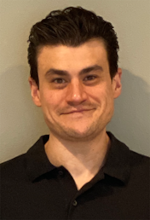
PI: Matthew Campbell, Ph.D., Acting Instructor, Department of Radiology
Project: Substrate metabolism linked redox-sensitive signaling mechanisms in mitochondria following exercise altered with age
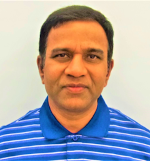
PI: Nagana Gowda, Ph.D., Research Associate Professor, Department of Anesthesiology & Pain Medicine
Project: Real time measurement of mitochondrial energetics in live muscle cells and isolate mitochondria
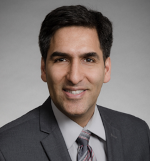
PI: Farid Moussavi-Harami, M.D., Assistant Professor, Division of Cardiology
Project: Computational and experimental methods to evaluate the role of sarcomeric variant incorporation in striated muscle
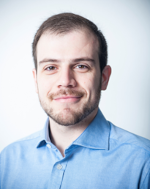
PI: Alec Smith, Ph.D., Acting Instructor, Department of Physiology & Biophysics
Project: Investigating the role of innervation in the development of hiPSC-derived skeletal muscle